
Our method is based on the rotationally invariant slave-boson framework, which is an efficient and reliable approach to study the electronic correlation effects in materials. Here, we develop an efficient theoretical approach for studying the two-particle response functions in strongly correlated systems, allowing for the investigation of arbitrary phase transitions induced from local electronic correlation effects.

However, the methodologies for calculating these response functions for strongly correlated materials are usually computationally intensive, hindering applications to material design. Two-particle response functions are essential for describing how electrons pair up in unconventional superconductors. Our approach paves the way for investigating the pairing mechanism in realistic correlated materials. On the other hand, the particle-hole spin fluctuations induce the s-wave pairing instability before entering Hund’s regime. By computing the pairing interaction considering the particle-particle and the particle-hole scattering channels, we identify the mechanism leading to the pairing instability around Hund’s metal crossover arises from the particle-particle channel, which contains the local electron pair fluctuation between different particle-number sectors of the atomic Hilbert space. The method is applied to the degenerate three-orbital Hubbard-Kanamori model for investigating the origin of the s-wave orbital antisymmetric spin-triplet superconductivity in Hund’s metal regime, previously found in the dynamical mean-field theory studies. Since there are 12 fundamental fermions, this would suggest that - if supersymmetry is true - there are another 12 fundamental bosons that have not yet been detected, presumably because they are highly unstable and have decayed into other forms.We develop an efficient approach for computing two-particle response functions and interaction vertices for multiorbital strongly correlated systems based on the rotationally invariant slave-boson framework. Bosonic Superpartners - Under the theory of supersymmetry, every fermion would have a so-far-undetected bosonic counterpart.The resulting theoretical particle is the graviton, which is predicted to have a quantum spin value of 2. Since the other fundamental forces - electromagnetism, strong nuclear force, and weak nuclear force - are all explained in terms of a gauge boson that mediates the force, it was only natural to attempt to use the same mechanism to explain gravity. Graviton - The graviton is a theoretical particle which has not yet been experimentally detected.
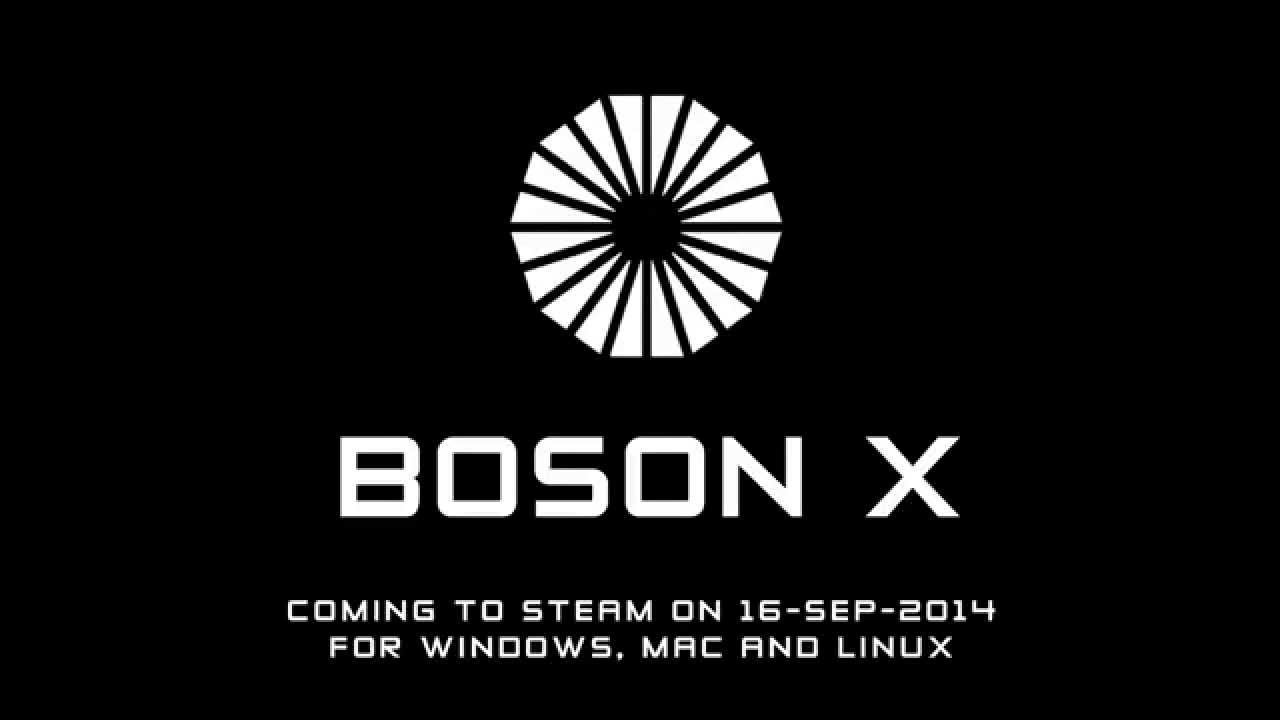
The particle is predicted to have a quantum spin value of 0, which is why it is classified as a boson. Further research is ongoing in an attempt to get better information about the particle's exact properties. On July 4, 2012, scientists at the Large Hadron Collider announced that they had good reason to believe they'd found evidence of the Higgs Boson.
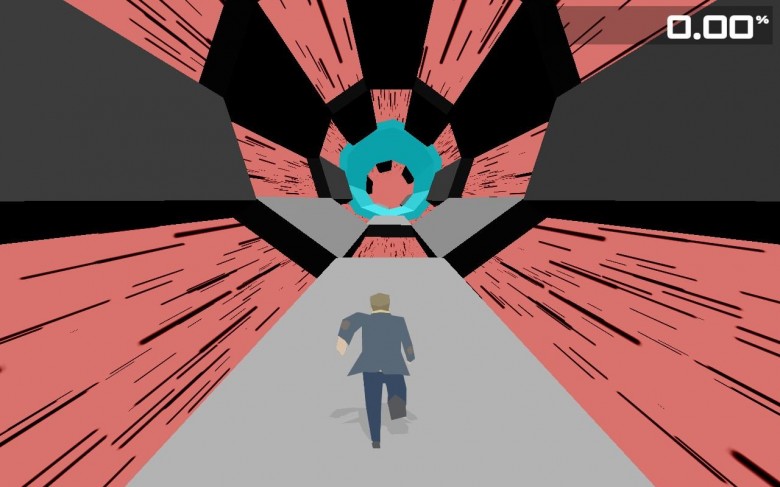
Higgs Boson - According to the Standard Model, the Higgs Boson is the particle that gives rise to all mass.
